Download: https://www.geoengineeringmonitor.org/2021/04/direct-air-capture.pdf
Description and purpose of the technology
Direct Air Capture (DAC) is a proposed Greenhouse Gas Removal (GGR) technology that some theorize could remove CO2 (and potentially other greenhouse gases) from the earth’s atmosphere on a large scale. In these proposals, the carbon is stored either underground through Carbon Capture and Storage (CCS) or in products of varying durability with through Carbon Capture, Use and Storage (CCUS) techniques.
DAC approaches use chemical reactions to scrub CO2 from the atmosphere, using substances able to act as a selective CO2 filter. The two most developed processes are liquid solvents and solid sorbents: CO2 dissolves in liquid solvent material, e.g. a strong hydroxide solution, or sticks to the surface of a solid sorbent, such as a plastic resin. Several DAC
concepts use large fans that move ambient air through the filters to enhance the capture process, because CO2 concentration in the atmosphere is in the parts permillion range.
The CO2 filtering process, however, is only the first step. To allow their repetitive use, the filters must be able to release the captured CO2. This
regeneration process typically requires high temperature (80°C to 800°C), which in turn requires high energy inputs.1 Additional DAC approaches include CO2 capture with battery-type devices, electrochemical reduction of CO2 or sorbents with humidity based release processes. The designs of the
proposed DAC plants range from shipping containers filled with CO2 collectors to artificial trees.2
All forms of DAC are extremely energy- and cost-intensive. The entire capture process for one tonne of CO2 requires between 5 to 10 GJ of
electrical and/or thermal energy.3 Cost estimates for DAC range from US$ ~100 to US$ ~1,000 per tonne, but lower costs for DAC have only been
proven theoretically.4 To have any significant effect on global CO2 concentrations, DAC would need to be rolled out on a vast scale, raising serious questions about the large amount of energy it requires, the levels of water usage for some technologies, land usage, and the toxicity impacts from the disposal of the chemical sorbents used. In addition, safe and long-term CO2 storage cannot be guaranteed.
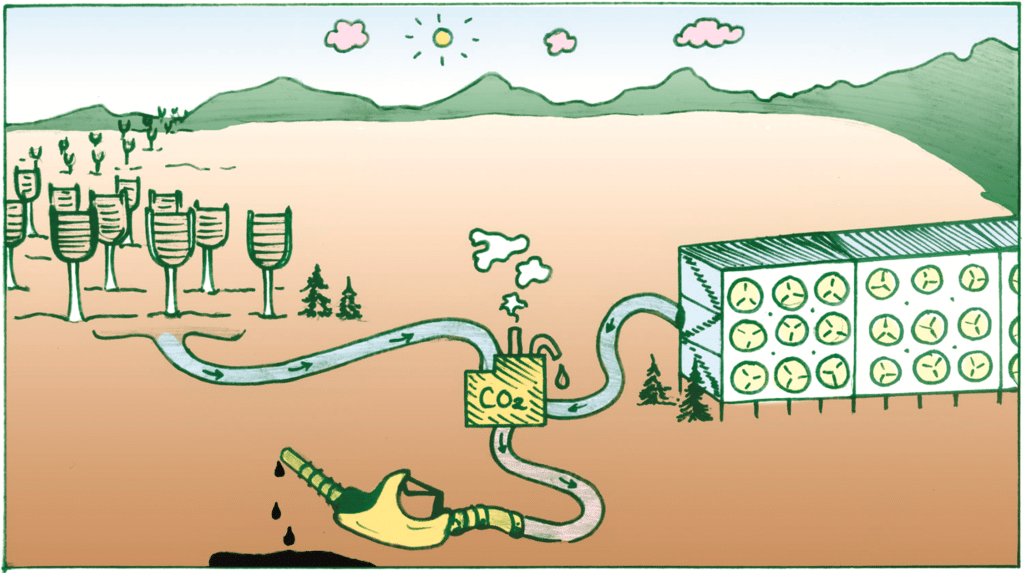
If a CCS approach is used, the captured CO2 is compressed into liquid form and transported to sites where it could be pumped into geological formations – theoretically for long-term storage, but that technology comes with a whole range of risks, among which leakage is an important one (see Technology Briefing on CCS).
CCUS is a proposal to “store” captured CO2 in goods with varying longevity, such as sparkling water, carbon-based fuels and chemicals, or building materials. The CO2, energy-intensively captured, usually re-enters into the atmosphere so it is at best a postponement of the emissions. (see Technology Briefing on CCUS).
The fossil fuel industry is attracted to DAC because the captured CO2 can be used for Enhanced Oil Recovery (EOR), which means more fossil fuels will be extracted and more CO2 is emitted.
All these techniques (CCS, CCUS, DAC) are especially of interest to fossil fuel industries, which are their main investors, because they help to justify continued extraction and use of dirty energy sources. This implies the continued devastation of poor communities around the world, with acute environmental justice, health and economic impacts, while having little evidence it can address the climate crisis at the scale required.
Actors involved
Several companies and research institutions are working to commercialize and advance DAC technologies. The sector receives private and public investments; double-digit million amounts are not uncommon.
The private investors include in particular, but not exclusively, the fossil fuel and mining sector, among them Australian oil company BHP, Bill Gates (who has major investments in oil transportation), the autoindustry, Chevron Technology Ventures, ExxonMobil, the Canadian tar sands mega-investor Murray Edwards, Occidental Petroleum and Shell. Most public funding to the DAC sector has been provided by the USA, the European Union (EU), Canada, Switzerland and Norway.5
Many DAC companies are spin-offs from research institutions.6 Climeworks AG, a spinoff of ETH Zürich, is the company with the most DAC plants so far.
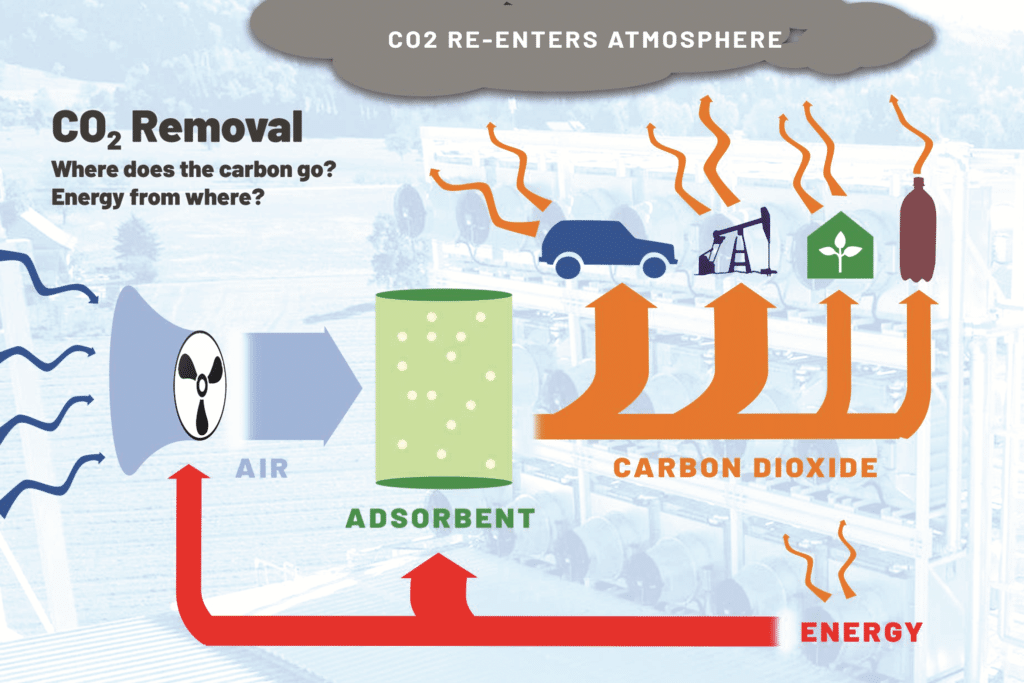
Impacts
Several companies and research institutions are working to commercialize and advance DAC technologies. The sector receives private and public investments; double-digit million amounts are not uncommon.
The private investors include in particular, but not exclusively, the fossil fuel and mining sector, among them Australian oil company BHP, Bill Gates (who has major investments in oil transportation), the autoindustry, Chevron Technology Ventures, ExxonMobil, the Canadian tar sands mega-investor Murray Edwards, Occidental Petroleum and Shell. Most public funding to the DAC sector has been provided by the USA, the European Union (EU), Canada, Switzerland and Norway.5
Many DAC companies are spin-offs from research institutions.6 Climeworks AG, a spinoff of ETH Zürich, is the company with the most DAC plants so far.
The company commissioned its first plant in 2017, in Hinwil, Switzerland and is participating in several research projects. According to Climeworks, the Hinwil plant captures about 900 tonnes of CO2 annually and delivers parts of the captured CO2 to a nearby greenhouse for CO2 fertilization. About 600 tonnes of CO2 are transported by truck to the Swiss production facility of Coca-Cola’s sparkling water brand “Valser”. Climeworks collaborates with several companies to develop and produce synthetic fuels manufactured from captured CO2, among them the Norwegian company Nordic Blue Crude AS as well as a joint project of the Italian oil group ENI and the Swiss spinoff Synhelion. Climeworks has been supported with more than € 50 million in public and private grants.7 Carbon Engineering Ltd., a company founded by David Keith (Harvard University) commissioned a pilot plant in Squamish, Canada in 2015, that captures about a tonne of CO2 a day. In 2017, the plant was connected to a fuel synthesis platform, aiming to produce synthetic transport fuels, based on the captured CO2 and hydrogen. The company has raised more than CAD 100 million from multiples private investors, including oil and mining companies, (Chevron, Occidental Petroleum and BHP) and public sources and plans to commission a larger DAC plant in cooperation with Oxy Low Carbon Ventures in 2023. The captured CO2 will be used for EOR, which means more fossil fuels and more CO2 emissions.
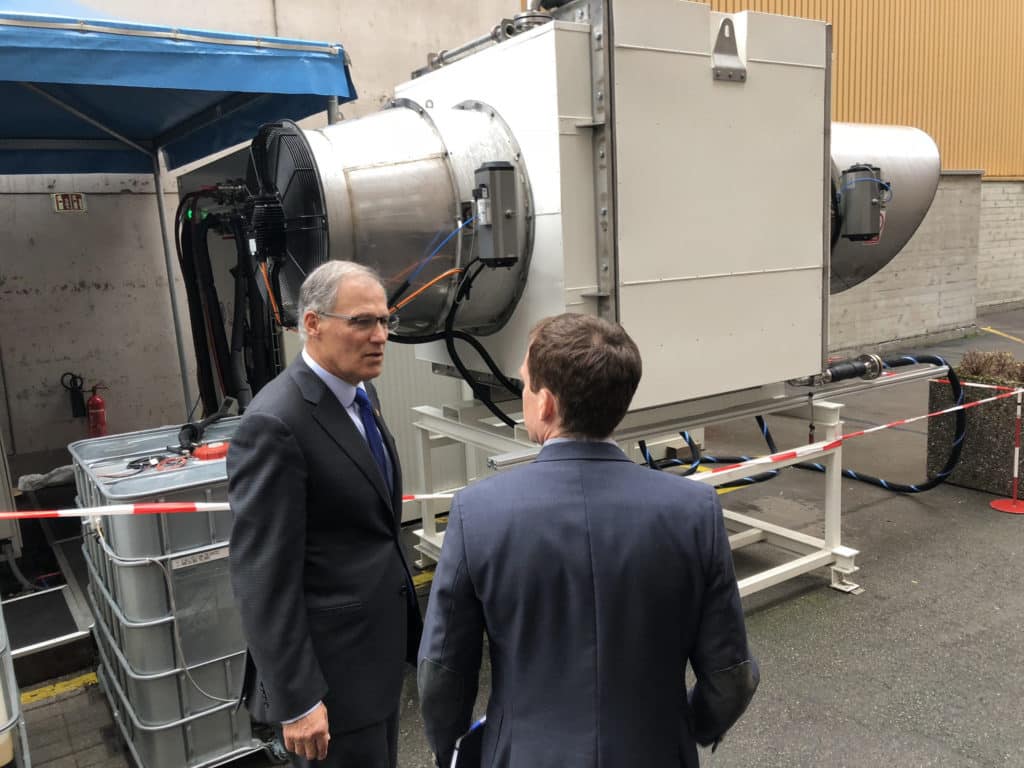
The US-based company Global Thermostat has operated a pilot DAC plant in Menlo Park, California, since 2010. In 2018, it opened its first commercial plant, capturing 4,000 tonnes of CO2 per year, in Huntsville, Alabama. In 2019, the company signed a joint development agreement with ExxonMobil to study the scalability of Global Thermostat’s DAC technology. As of this writing, the company has raised more than US$ 70 million in funding.9
Worldwide, there are more than ten other initiatives to further develop and market DAC technology, among them the Finnish Soletair Power. The company developed a technology that combines DAC, an electrolyser for hydrogen production, and a synthesis reactor for hydrocarbon production and commissioned its first demonstration facility in 2018. The US based company Infinitree LLC develops a CO2 capture system for use in greenhouses.
The Dublin-based company Silicon Kingdom Holdings plans to commercialize DAC technology developed at the Arizona State University’s Centre for Negative Carbon Emissions and plans to “plant” 1,200 mechanical trees for CO2 capture.10
The world’s largest research program on DAC is the EU-funded pan-European research project CarbFix, led by Reykjavik Energy. The project combines DAC with CCS and involves capturing CO2 and H2S at Reykjavik Energy’s Hellisheidi Geothermal Power Plant, nearby Reykjavik. The CO2 is dissolved in water under pressure, and the solution is injected into basaltic formations nearby the plant, at 400 m to 800 m depth, with the aim of storing the gas in mineral form in the bedrock. The European follow-up project GECO (Geothermal Emission Control) is conducted to deepen and further develop the CarbFix results at five demonstration sites throughout Europe.11
Impacts of the technology
The main problem with DAC, as with CCS and CCUS (all especially of interest to fossil fuel industries, which are their main investors), is that it prolonges the life of dirty energy sources and the continued devastation of poor communities around the world. They cause acute environmental justice, health and economic impacts. There is no evidence that these technologies can address the climate crisis at the scale required.
Although little is known about the CO2-capture efficiency, safety and impacts of DAC, several companies have already started marketing DAC as a climate solution. Whether the technology itself is climate friendly needs to be critically questioned, among other reasons, because DAC requires considerable energy inputs. These inputs produce GHG emissions if not fully sourced from renewable energy. The energy required to capture the 600 tonnes of CO2 provided by Climeworks to the sparkling water brand “Valser” is sufficient to supply 760 EU citizens with electricity for a period of one year, although this number excludes the energy needed to compress, purify and transport the CO2 over ~200 km by truck.13
With many people – particularly in the Global South – still without access to electricity, it seems incompatible with principles of global climate justice to use excessive amounts of renewable energy capacity on Northern developed countries that will help the polluting industries continue business-as-usual. Deployed on full-scale, DAC plants require significant infrastructure. To capture one million tonnes of CO2 annually in a liquid solvent system, the land footprint has been estimated at 60 to 100 km² for a system powered by solar energy. This means that operating DAC at a scale sufficient to make an impact on global carbon emissions would be a considerable threat to large areas of natural ecosystems.14 A full life-cycle analysis on the construction, maintenance and environmental impacts of largescale DAC plants is not available and is a serious knowledge gap. For example, little is known about the toxicity, production and disposal of the CO2 solvents and sorbents in use.
The hydroxide solutions in use, e.g. Carbon Engineering’s potassium hydroxide solution, require substantial amounts of energy and water during production, and are highly corrosive. Leaks during the capture cycle may occur.15 Water consumption is also an issue during the DAC process: the water consumption for one tonne of CO2 captured is estimated at 5 to 13 tonnes of water and some solid sorbent based DAC processes may require up to 20 tonnes of water per each tonne of CO2 captured.16 Scaled to climate relevant dimensions, this technology could exacerbate water scarcity – which is already one of the serious problems of the climate crisis.
The captured CO2 is proposed as a feedstock for CCS or for industrial uses. DAC proponents trust that geological storage of CO2 in empty oil and gas reservoirs, or in deep saline aquifers, will be available, effective and reliable.
Yet there is little real-world experience on which to base that faith. It appears unlikely that geological storage can ever guarantee reliable and durable storage – even before storing billions of tonnes of carbon is discussed. In 2018, a group of authors argued that the injections at the Icelandic DACCS site Hellisheidi led to induced seismic activity.17
Using captured CO2 for EOR leads to even more fossil fuel emissions. In cases where the captured CO2 is used in consumer products (CCUS), it usually re-enters into the atmosphere and the very likely overall result is that more CO2 ends up in the atmosphere than is actually removed due to the large amounts of energy used for the DAC process.
A modelling exercise looking at the impact of DAC on climate stabilization efforts predicted that it would postpone the timing of mitigation (emissions reductions) and allow for a prolonged use of oil, impacting positively on energy exporting countries.18 This is of course similar for many geoengineering technologies and manufactured consent for further fossil fuel use is one of the main dangers DAC and other Carbon Capture schemes pose.
Reality check
The engineering approaches for DAC diversified and there are more than twenty trial sites, but none are operating at a commercial scale. Larger-scale DAC sites have been announced and numerous new research projects are underway. A massive scale-up of DAC is only achievable with a major increase in energy production, massive amounts of water, and considerable amounts of funding. The fate of the captured CO2, and whether there will be any permanent storage options, remains highly uncertain.
Further reading
Geoengineering Monitor (2019), “Direct Air Capture – recent developments and future plans”
https://www.geoengineeringmonitor.org/2019/07/direct-air-capture-recent-developmentsand-future-plans/
ETC Group and Heinrich Böll Foundation, “Geoengineering Map” https://map.geoengineeringmonitor.org/
Endnotes
- Beuttler, et al. (2019) The Role of Direct Air Capture in Mitigation of Anthropogenic Greenhouse Gas Emissions, in: Front. Clim., published online: November 21, 2019, https://www.frontiersin.org/articles/10.3389/fclim.2019.00010/full; ETC Group and Heinrich Böll Foundation (2020) Geoengineering Map, https://map.geoengineeringmonitor.org/
- ETC Group and Heinrich Böll Foundation (2020)
- National Academies of Sciences, Engineering, and Medicine (2019) Negative Emissions Technologies and Reliable Sequestration: A Research Agenda, Washington, DC: The National Academies Press, 510 pages, ISBN 978-0-309-48452-7, https://doi.org/10.17226/25259; Gambhir & Tavoni (2019) Direct Air Carbon Capture and Sequestration: How It Works and How It Could Contribute to Climate-Change Mitigation, in: One Earth, Vol. 1(4): 405-409
- Nisbet (2019) THE CARBON REMOVAL DEBATE. Asking Critical Questions About Climate Change Futures, Carbon
Removal Briefing No. 2, Institute for Carbon Law Removal and Policy, American University, 24 pages, https://www.american.edu/sis/centers/carbon-removal/upload/carbon-removal-debate.pdf; Fuss, et al. (2018) Negative emissions-Part 2: Costs, potentials and side effects, in: Environmental Research Letters, Vol 13(6): 063002,
https://iopscience.iop.org/article/10.1088/1748-9326/aabf9f - ETC Group and Heinrich Böll Foundation (2020)
- ETC Group and Heinrich Böll Foundation (2020)
- ETC Group and Heinrich Böll Foundation (2020)
- ETC Group and Heinrich Böll Foundation (2020)
- ETC Group and Heinrich Böll Foundation (2020)
- ETC Group and Heinrich Böll Foundation (2020)
- ETC Group and Heinrich Böll Foundation (2020)
- ETC Group and Heinrich Böll Foundation (2020)
- Eurostat (2019) Electricity and heat statistics, accessed: February 2020, https://ec.europa.eu/eurostat/statisticsexplained/index.php/Electricity_and_heat_statistics#Consumption_of_electricity_per_capita_in_the_households_sector; ETC Group and Heinrich Böll Foundation (2020)
- National Academies of Sciences, Engineering, and Medicine (2019); Nisbet (2019)
- Realmonte, et al. (2019) An inter-model assessment of the role of direct air capture in deep mitigation pathways,
Natural Communications, Vol. 10, https://www.nature.com/article s/s41467-019-10842-5; National Academies of
Sciences, Engineering, and Medicine (2019) - Realmonte, et al. (2019)
- Juncu, et al. (2018) Injection-induced surface deformation and seismicity at the Hellisheidi geothermal field, Iceland, Journal of Volcanology and Geothermal Research, Vol. 391, https://www.sciencedirect.com/science/article/pii/S0377027317304080?via%3Dihub
- Chen and Tavoni (2013) Direct air capture of CO2 and climate stabilization: A model based assessment, Climatic
Change, Vol. 118: 59–72, https://doi.org/10.1007/s10584-013-0714-7